Glucose oxidation is a chemical process that provides energy for an organism to carry out all of its required activities. During this process, glucose, a simple sugar molecule obtained from food, is broken down into carbon dioxide and water. This reaction releases energy and stores it in a chemical form for the cell to use. There are three separate stages of glucose oxidation: glycolysis, the citric acid cycle, and the electron transport system.
Glucose
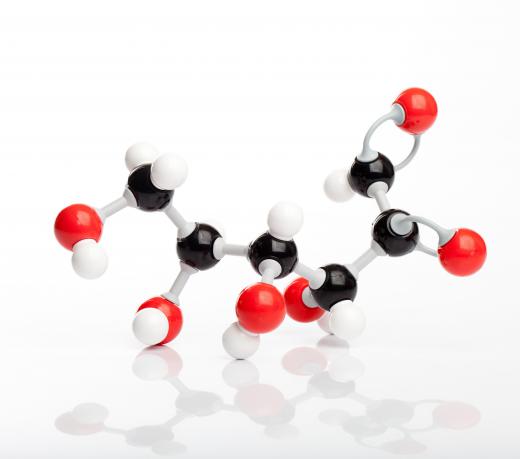
Molecules of glucose are used to build more complex carbohydrates, like starch and cellulose. The chemical formula for this molecule is C6H12O6, meaning that it is made up of six carbon atoms, 12 hydrogen atoms, and six oxygen atoms. Found in plants and many types of food, glucose is absorbed into the bloodstream during digestion.
Oxidation
Glucose oxidation is an aerobic process, a chemical reaction that requires oxygen. The term "oxidation," in fact, refers to any reaction where oxygen is combined with another molecule, which is then said to be oxidized. During the process, one glucose molecule combines with six oxygen molecules to produce six carbon dioxide molecules, six water molecules, and adenosine triphosphate (ATP), a molecule that cells use to store or transfer energy.
Glycolysis
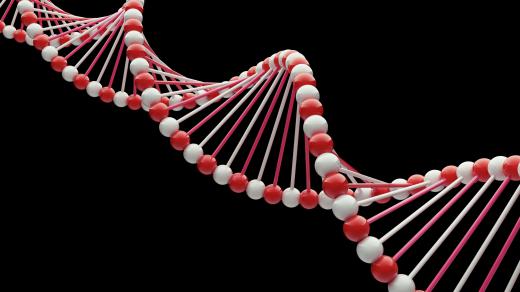
The first step in the oxidation process is glycolysis, which takes place within a cell’s cytoplasm, the gel-like substance that fills the cell and surrounds the other cellular organs. During this stage, the glucose molecule is broken down into two molecules of pyruvate, an organic acid that can supply cells with energy. This breakdown also releases energy, which is used to add a phosphate ion to adenosine diphosphate (ADP) to create ATP. ADP, in turn, is formed with ATP is broken down to release its energy.
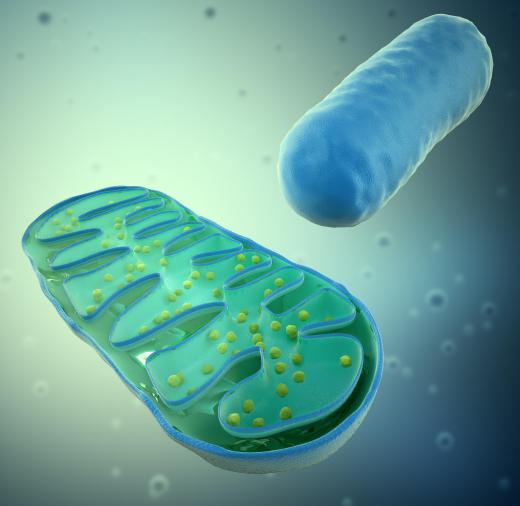
Glycolysis of a single glucose molecule consumes two ATP molecules, and produces four in total, leading to a net energy gain of two ATP. The energy from the process is also used to produce two NADH, a form of an enzyme used to transfer electrons to power cellular chemical reactions.
The Citric Acid Cycle
To begin the citric acid cycle, also called the Krebs cycle, pyruvate molecules produced by glycolysis are moved to the mitochondria, a cellular organ involved in metabolic processes. Once there, the molecules are converted into acetyl CoA, the molecule that powers the citric acid cycle. Acetyl CoA is made up of carbon from the pyruvate and coenzyme A, a molecule that assists in biological processes. The conversion process produces one NADH.
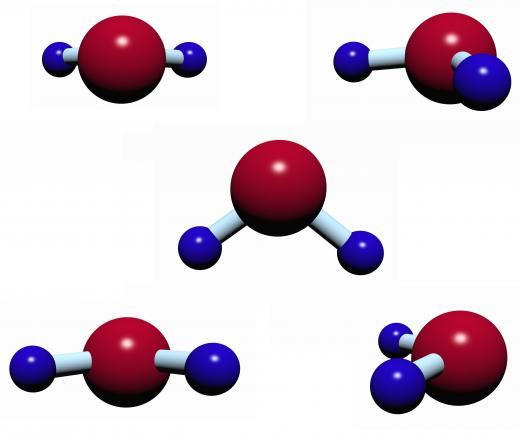
Acetyl CoA releases the carbon portion of the molecule into the citric acid cycle, which runs constantly, producing ATP, high energy electrons, and carbon dioxide. Most of the energy produced is stored in the form of high energy electrons, and one turn of the cycle will result in three NADH and one FADH2. Like NADH, FADH2 stores the captured electrons. The cycle also produces two ATP, and gives off the rest of the energy as heat.
The Electron Transport System
The final stage of glucose oxidation also takes place within the mitochondria, where a group of proteins, called the electron transport system, help transform the energy of the electrons captured by NADH and FADH2 into ATP. This process is modeled by chemiosmotic theory, which describes the way these electrons pass along the transport system, releasing energy as they move through.
The released energy is used to move positively charged hydrogen ions back and forth across the membrane separating two parts of the mitochondria. Energy from this movement is stored in ATP. This process is called oxidative phosphorylation, because oxygen is necessary for the final step, accepting electrons and hydrogen atoms to become H2O, or water. The energy yield from this stage is 26 to 28 ATP.
Energy Gained
When a single molecule of glucose is oxidized, the cell gains about 30 to 32 ATP. This number can vary, because often a mitochondrion does not work at full capacity. Some energy may be lost as the NADH molecules formed in glycolysis transfer their electrons through the membrane separating the mitochondria and the cytoplasm.
ATP
ATP is present in all living organisms and plays a critical role in cell metabolism, as it is the main way cells store and transfer energy. Plants produce it by photophosphorylation, a process that converts sunlight to energy. ATP can also be produced in an anaerobic process, a reaction that does not require oxygen. Fermentation, for example, can take place with no oxygen present, but this and other anaerobic metabolic processes tend to be much less efficient ways of making this molecule.
A large number of cellular functions require ATP. The cell breaks down these molecules into ADP and phosphate ions, releasing the stored energy. This energy is then used to do things like move large molecules in and out of the cell or to help create proteins, DNA, and RNA. ATP is also involved in muscle movement and is essential for maintaining the cell’s cytoskeleton, the structure within the cytoplasm that supports the cell and holds it together.